How radar and surveillance technologies are evolving for modern security and defense
From AESA radars to AI-powered video analytics, modern surveillance technology is undergoing rapid transformation. This post explores how advances in RF, digital processing, and multi-sensor fusion are shaping the future of security, counter-stealth detection, and threat monitoring.
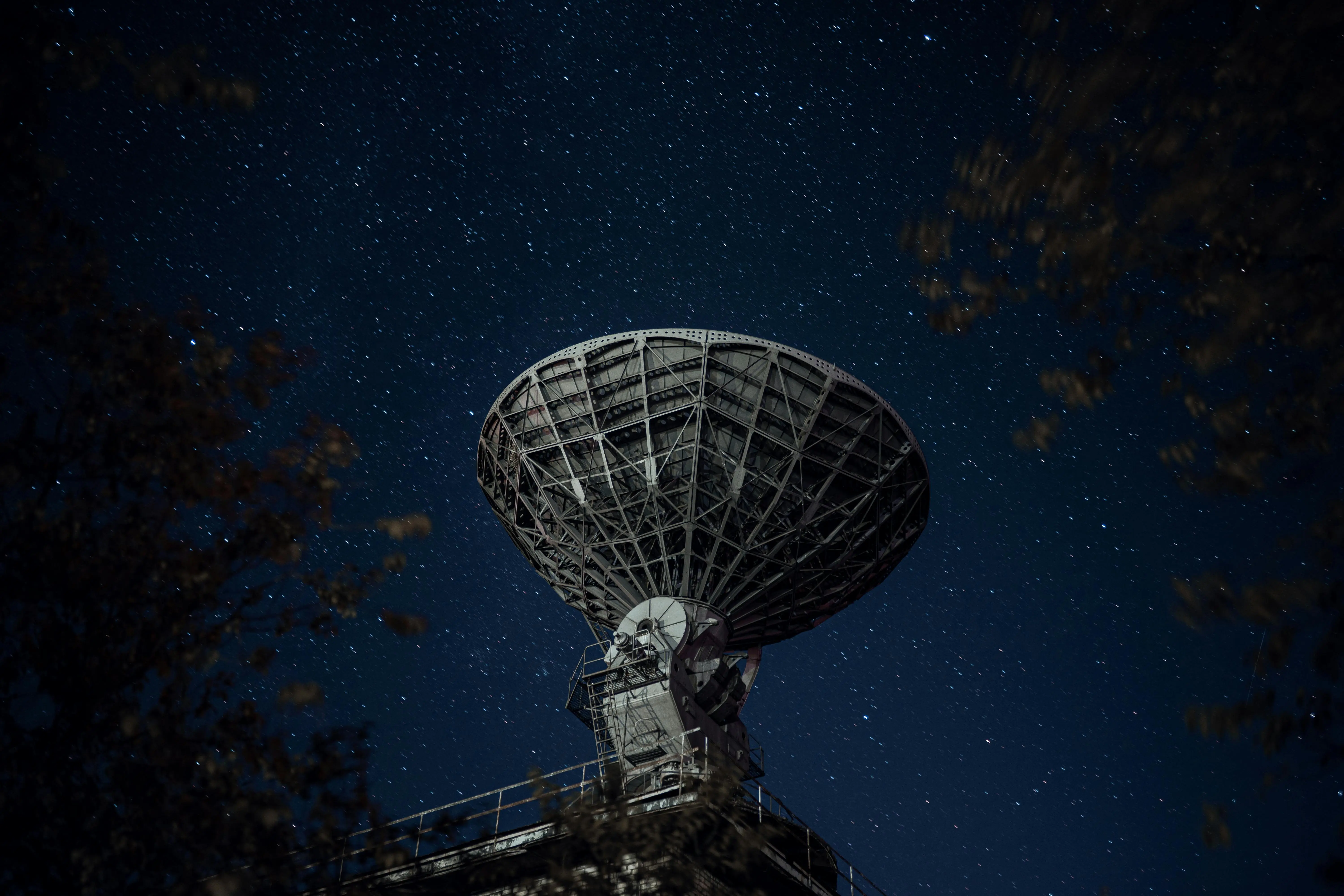

Eliza Miller
March 21, 2025
Modern radar and surveillance systems are undergoing a renaissance, driven by new security challenges and enabled by cutting-edge technologies. Today’s threats are more varied and technically advanced – from stealth aircraft and hypersonic missiles that are hard to detect, to swarms of small drones that overwhelm traditional sensors, and even to asymmetric threats in civilian settings that demand smarter surveillance. In response, radar and sensing technology is evolving rapidly. Active electronically scanned arrays, digital signal processing, artificial intelligence, and sensor fusion are among the innovations redefining how we achieve persistent situational awareness. These trends are ensuring that radar and surveillance capabilities keep pace with the demands of national defense and public security in the 21st century.
A modern naval radar mounted on a warship. Advanced radar systems like AESA arrays are crucial for tracking multiple threats and operating in contested electromagnetic environments. They exemplify how defense surveillance tech is evolving to meet new challenges.
Active Arrays and High-Power Electronics (GaN)
One of the most significant leaps in radar tech has been the wide adoption of Active Electronically Scanned Arrays (AESA). Unlike traditional dish radars that mechanically rotate a single beam, AESA radars use a matrix of many small antenna elements, each with its own transmit/receive module, to electronically steer multiple beams. This provides instant beam agility, the ability to track numerous targets simultaneously, and improved reliability (if one element fails, the radar still operates). A key enabler for AESA has been the maturation of compound semiconductor components, especially Gallium Nitride (GaN) power amplifiers. GaN can handle higher voltages and power with greater efficiency than previous technologies, allowing radars to transmit stronger signals or run cooler. NI (National Instruments) notes that GaN is considered “the biggest semiconductor innovation since silicon” for RF front-ends, significantly benefiting AESA radar evolution. With GaN-based T/R modules, AESA arrays achieve more power on target and longer range without requiring bulky cooling systems. For example, an older generation array might have used gallium arsenide (GaAs) components and needed large heat sinks, whereas a GaN-based AESA of similar size can output equivalent power in a smaller form factor and with less cooling.
The net effect is that today’s frontline radars – on fighter jets, naval ships, and ground systems – are far more capable. They can interleave modes (e.g., one radar performing surveillance, target tracking, and missile guidance simultaneously by time-sharing the array), have improved resolution and jam-resistance, and can even adapt their beam patterns to the environment in real time. For instance, AESA radars can employ low probability of intercept waveforms and rapidly null out jamming sources by placing nulls in the antenna pattern. The U.S. Army’s new WiSPR system (Wideband Selective Propagation Radar) demonstrates some of these capabilities: it’s a multi-function AESA that operates at novel frequencies with inherent low probability of detection, designed to counter adversary sensors while avoiding detection itself. WiSPR even doubles as a communications system, highlighting how versatile modern arrays have become. After years of development, its LPI/LPD features and integration into active protection systems are now moving into production
Digital Signal Processing and Cognitive Radar
As important as the RF hardware is, the digital side of radar has seen equally transformative innovation. Advances in high-speed ADCs, DACs (as discussed earlier), and embedded processing allow more of the radar’s functionality to be defined in software – spawning the era of digital and cognitive radar. Cognitive radar refers to systems that can dynamically adapt their operating parameters (waveform, frequency, pulse repetition, etc.) based on the environment and mission objectives, using feedback and machine learning to optimise performance in real time.
This concept, pioneered in research, is moving toward deployment thanks to powerful FPGAs and AI processors embedded in radar signal processors. Modern FPGAs, for example, provide the computational throughput to handle machine learning algorithms directly in the radar signal path, something not possible just a decade ago. An adaptive radar might, for instance, recognise that a certain target is maneuvering and switch to a waveform better suited for tracking it, or detect that there’s heavy interference in part of the band and hop to a clearer frequency – all autonomously. The NI radar technology review specifically highlights “machine learning techniques within cognitive radar” made possible by new FPGA tech, enabling features like automatic target recognition (ATR) and knowledge-aided waveform selection. Instead of pre-programmed modes (search, track, etc.), the radar can fluidly choose the best mode for the situation, which is a game-changer for complex scenarios like cluttered ground environments or electronic attack conditions.
AI is also revolutionising video and imagery surveillance. Beyond radar, optical surveillance cameras are now often augmented with AI analytics for object detection, facial recognition, and behaviour analysis. This helps security personnel handle the deluge of video feeds by automatically flagging unusual activities. The surveillance industry is moving toward “proactive video security enabled by artificial intelligence,” where cameras (or cloud systems processing their feed) trigger real-time alerts and filter out false positives. For example, an AI-enabled camera network in a city can alert authorities to a crowd forming in a sensitive area or detect a person carrying a weapon, in ways that older CCTV setups never could. The market for AI in video surveillance is booming, with expected growth over 20% CAGR through 2030, underlining how integral AI has become to surveillance trends.
Multi-Static Sensors and Sensor Fusion
Another major trend is the use of distributed and multi-static sensor systems. Instead of one monolithic radar doing all the work, multiple smaller sensors networked together can cover an area more effectively and resiliently. Passive radar is one example – it involves receivers that use third-party transmissions (like commercial broadcast signals or even other radars) reflected off targets, rather than emitting their own signal. This makes the radar system essentially invisible and immune to anti-radar weapons. European defense firms have been investing in passive radar as part of the push for spectrum superiority; a Leonardo publication notes that passive systems exploit “existing sources (e.g., broadcast signals) for detection” and are inherently LPI. Such systems complement active radars by filling coverage gaps and providing covert surveillance.
Similarly, multi-static radar setups, where multiple spatially separated transmitters and receivers work collaboratively, are gaining attention (Fraunhofer FHR in Germany is even hosting focus days on “Multistatics and Passive Radar” in 2025 to showcase research). Multi-static configurations can be harder to jam (an adversary would have to jam multiple geometrically diverse paths) and can improve target detection of stealth objects via geometric diversity (a stealthy target might evade one radar aspect but be seen by another).
Hand in hand with multiple sensors is the idea of sensor fusion in surveillance. The F-35 fighter famously aggregates data from numerous onboard sensors – radar, IR sensors, EW receivers – into a unified picture for the pilot. This is enabled by very high bandwidth data buses and processing that can combine these feeds. The benefit is a more robust detection: one sensor can validate another or add attributes (e.g., radar gives range and velocity, infrared search and track gives angle and perhaps classification). On the ground, we see fusion between video surveillance and radar in systems guarding critical facilities: ground surveillance radars detect movement or intrusions at long range, and cue PTZ (pan-tilt-zoom) cameras to zoom in and identify the object. Each sensor on its own has limitations (radar might see a blob, camera can identify a person but only at closer range), but together, they provide a powerful surveillance capability.
Underpinning fusion is the need for high-speed interconnects and common data models. Modern C4ISR (Command, Control, Communications, Computers, Intelligence, Surveillance, and Reconnaissance) systems emphasise open architectures so that sensors from different manufacturers can share data. Standards like the USA’s Sensor Open Systems Architecture (SOSA) are pushing this forward.
Meeting New Security Challenges
These technological trends – AESA with GaN, cognitive radar with AI, passive/multi-static deployments, and sensor fusion – are largely motivated by specific emerging security challenges:
- Stealth and Counter-Stealth: Stealth aircraft and missiles are designed to be hard to detect on conventional radars. AESA radars with broad bandwidth and agile waveforms help counter stealth by operating across frequencies (a target optimised to evade X-band might be seen in L-band, for example) and by using tailored waveforms. Passive and multi-static radars add further counter-stealth capabilities, as a stealth platform can’t mask its reflections from all directions simultaneously.
- Drone Swarms and Low Slow Targets: Small drones present a difficult target – low radar cross-section, slow-moving like birds, often flying low. Traditional high-power radars may struggle with these due to ground clutter and resolution limits. The solution is multi-sensor: high-resolution short-range radars, optical trackers with AI classification (to tell drone apart from bird), and even RF detectors to pick up control signals. We see the market responding with counter-UAS systems that combine 3D radar, EO/IR cameras, and jamming interdiction.
- Electronic Warfare (EW) and Spectrum Contested Environments: Potential adversaries will try to jam or spoof our sensors. Cognitive techniques and robust waveforms (like frequency hopping, direct sequence spread spectrum) are a countermeasure. For instance, the Army WiSPR’s use of wideband novel modulations with LPI characteristics is specifically to operate under heavy SIGINT and EW threats. Additionally, testing and evaluation now routinely involve robustness against electronic attack, leading to radar test equipment that can simulate dense jamming and false targets. The emphasis is on radars that can not only detect targets but do so while being bombarded by interference – and even use that interference to the radar’s own advantage when possible (e.g., geolocating a jammer).
- Persistent Surveillance and Big Data: On the civilian side, preventing terrorist attacks or managing large events requires analysing vast amounts of surveillance data. The trend toward AI and cloud-connected cameras directly addresses this by enabling proactive surveillance – identifying threats (a person with a weapon, an unattended bag, a license plate on a watchlist) in real time rather than just recording for after-the-fact analysis. Cloud computing and IoT integration means surveillance cameras are no longer isolated; they feed into integrated command centers where data is correlated with other sources (social media alerts, police databases), enhancing overall situational awareness.
In summary, radar and surveillance technology is rapidly innovating to meet both traditional military threats and modern security needs. The combination of advanced hardware (like GaN-based AESAs) and advanced software (AI-driven signal processing) yields systems that are more flexible, powerful, and intelligent than their predecessors. As threats continue to evolve – faster, smaller, stealthier, more proliferated – the surveillance systems tasked to detect them are keeping pace by becoming more adaptive and networked. We are entering an era where a “radar” is not a standalone rotating dish, but a sophisticated networked sensor node, possibly one of many, leveraging massive computing power and smarts. These innovations ensure that whether it’s guarding a nation’s skies or securing a city’s streets, we can maintain the sensor advantage required for timely and effective response to security challenges.
Sign up for expert insights on cutting-edge technology
Stay informed on innovations that power the future, directly from our team to your inbox.